A brief introduction to molecular spectroscopy
The atoms that make up a molecule are not immobile, but oscillate around their equilibrium position. These oscillations are grouped according to how they change the molecular geometry and are called vibrational modes.
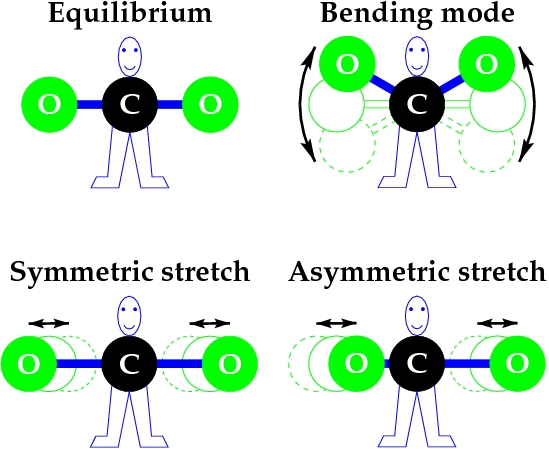
An illustration of the fundamental vibrational modes in the carbon dioxide (CO2) molecule.
The number of possible vibrational modes depends on the exact molecular geometry and the number of atoms in the molecule. At the same time, the molecules can also rotate around their various symmetry axes.
In quantum mechanics, these vibrations and rotations are quantized, that is, the atoms can only oscillate or rotate at very specific frequencies and those frequencies are characterized by quantum numbers; the vibrational quantum states are generally denoted by v; the rotational quantum states generally by J. To go from a certain state to a higher one, the molecule needs to absorb a photon with exactly the right energy; conversely, to go to a lower state the molecule will emit such a photon. For vibrational states, these photon energies are typically in the infrared whereas for pure rotational states, the photon energies are much lower and typically lie in the microwave part of the spectrum. Since the rotational properties however depend on the geometry, each vibrational state has its own set of rotational states.
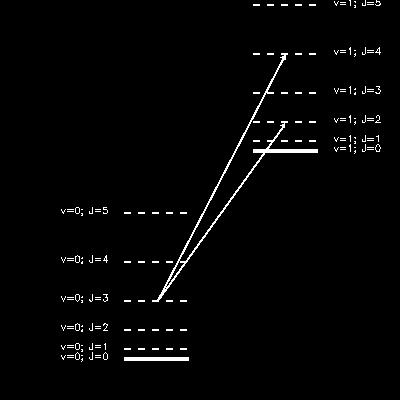
An illustration of how rotational and vibrational quantum states are organized. The left part of this diagram corresponds to the rotational levels (denoted by their quantum number J) in the vibrational ground state (v=0). The next higher vibrational state (v=1) has its own set of rotational quantum states. Because of selection rules, a vibrational transition generally also involves a change in rotational quantum state, illustrated by the arrows.
Due to selection rules, vibrational transitions generally also
involve a rotational transition. Therefore, unlike atoms, molecular
spectra show tremendous structure in their absorption and emission
bands, caused by the superposition of many individual transitions that
are due to changes in the vibrational and rotational states. When
observed at high spectral resolution, these individual transitions are
clearly visible; at lower spectral resolution the transitions blend
into a broader ro-vibrational band. Since the vibrational and
rotational parameters are different for each molecule, these bands are
a unique fingerprint and can be used to reliably identify the presence
of molecules in astronomical objects. This is often of great
importance for understanding the chemistry that occurs in
astrophysical environments.
In most cases, the exact shape of the molecular bands is moreover very
sensitive to the local temperature and density. Therefore, if we
understand e.g. how the band profile changes with temperature, we can
use the molecular bands as distant thermometers and measure the
temperature in the same astrophysical environments. The molecular
bands can thus be used to study at the same time the chemistry and the
physical conditions in many astronomical objects.
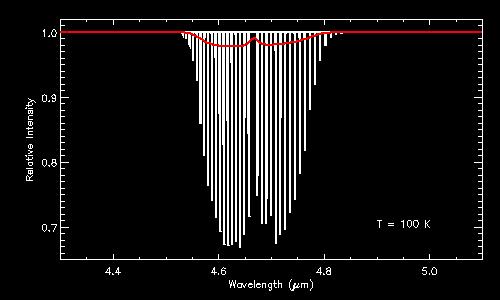
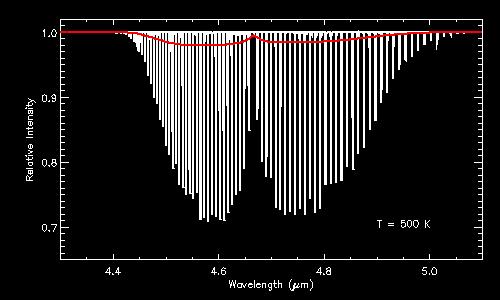
Some illustrations of ro-vibrational bands. Both figures are infrared absorption spectra of CO (carbon monoxide). The top panel shows the absorption spectrum at a temperature of 100 K; the bottom panel at 500 K. In both panels, the individual lines that make up the ro-vibrational band are clearly visible in the high-resolution spectra (in white), whereas this information is lost in the low-resolution spectra (in red). Notice how the profile of the band changes with temperature. Even at low resolution, it is thus possible to infer the temperature by studying the shape of the band profile.